Project
A new approach to fight against lung fungal pathogens
Fungal infections are estimated to kill more than 1.5 million people worldwide per year. However, the issue of fungal pathogenicity is largely neglected. Fighting fungal pathogens poses a series of unique challenges: timely diagnosis is difficult, the existing arsenal of antifungal drugs is limited, and the effectiveness of the current antifungal therapies has plateaued, particularly due to the emergence and spread of resistance. In addition, life threatening fungal infections usually occur in the context of significant co-morbidities. As the number of people at risk is rising, it is urgent to find new approaches that aim to modify the host-pathogen interactions.
This project focuses on lung infection caused by Aspergillus spp. (the most frequently isolated filamentous fungus in humans) in a complementary way to traditional pharmaceutical drug discovery, using “environmental interference”. This innovative approach exploits the ecology of the lung microbiota, and more concretely, the ability of airway bacteria to consume oxalic acid required by the pathogen during infection, as one of the cornerstones to control the growth of Aspergillus spp..
We will translate the promising results obtained in artificial lung cell cultures into an in vivo model to reach a status of a pre-clinical trial. Secondly, we will produce a new collection of airway bacteria with the ability to control Aspergillus spp. via oxalate consumption. Finally, we will screen the lung microbiome for the early identification of at-risk patients. This unique approach is made possible by a collaboration of bioinformaticians, microbial ecologists and clinicians, linked to two Swiss companies that are pioneers in the field of cell cultures and organs on chip. The results obtained across a wide range of experimental approaches will provide a unique toolset for personalized management and treatment of life-threatening aspergillosis.
Fungal pathogenesis
One of the most challenging public health issues the world is currently facing is the so-called antibiotics crisis, which refers to the prevalence of pathogens resistant to most of the available antibiotic compounds in medical use. While the risk of untreatable bacterial infections has been widely acknowledged, an equally concerning problem involves an increasing rate of resistance among opportunistic fungal pathogens. Fungal diseases are estimated to kill more than 1.5 million people per year, with the number of affected patients approaching a billion. Despite this, the issue of fungal pathogenesis has been largely neglected.
The chemical arsenal of existing antifungal drugs is very limited, and the same classes of molecules are used in both agriculture and human health. For instance, the frontline antifungal drugs, azoles, are used in crop protection and in human and animal health, leading to the emergence and spread of resistance among pathogens.
Most human fungal infections are caused by well-known airborne fungal opportunists from the genera Aspergillus, Cryptococcus, Histoplasma and Blastomyces, and also by the commensal yeast Candida albicans.
Pulmonary aspergillosis: incidence and treatment
Aspergillus is the most frequently isolated filamentous fungus in humans and animals. Aspergillus spp. are associated with a variety of clinical manifestations ranging from allergic disease to life-threatening systemic infections. Lung infections due to Aspergillus spp. are caused by inhalation of airborne conidia (fungal spores). Concentration of conidia in the air range from 1 to 100 per m3, but can reach up to 108 per m3 in some environments. Inhaled conidia are usually eliminated by neutrophils and macrophages (innate immune system) in immunocompetent individuals. However, depending on the virulence of the fungal strain, as well as the immunological status and the pulmonary structure and function of the host, Aspergillus can lead to a variety of pathologies. In view of the variability in pathogenicity within Aspergillus, there is an urgent need to understand the conditions that make the respiratory tract permissive to conidial germination in susceptible individuals, and in particular, to determine whether the composition of the airway microbiota plays a role in this regard.
The spectrum of Aspergillus spp. pulmonary manifestations is wide and includes colonization (i.e. presence of fungi without clinical symptoms or signs of active disease), infection (chronic aspergillosis, invasive aspergillosis) or hypersensitivity responses (asthma, allergic bronchopulmonary aspergillosis – ABPA). These manifestations depend largely on the immune status of the host. Aspergillus spp. can lead to a chronic, non-invasive form of infection called chronic pulmonary aspergillosis (CPA). The global burden of CPA is estimated to more than 350’000 cases per year. Invasive pulmonary aspergillosis (IPA) is the most severe and lethal form of Aspergillus infection occurring mainly in immunosuppressed patients. The incidence of IPA is approximately 250’000 cases annually and the mortality rate ranges from 30 to 80%.
On the other side of the spectrum, Aspergillus spp. can cause hypersensitivity responses. In the atopic patient, the most severe form of aspergillosis is the allergic bronchopulmonary aspergillosis (ABPA). ABPA affects close to 5 million patients worldwide.
The current available treatments are highly limited. The primarily treatment in the case of CPA or IPA is the use of triazoles such as voriconazole or itraconazole. Surgical resection is reserved for patients presenting associated complications such as severe hemoptysis. Corticosteroids are the mainstay of ABPA treatment with or without antifungal agents.
Importance of oxalate in Aspergillosis
Fungi of the genus Aspergillus are widespread in the environment and are commonly isolated from both outdoor and indoor environments. Aspergillus spp. can use multiple organic substrates and adapt to a broad range of environmental conditions.
Although many aspects of the ecology of Aspergillus spp. have been investigated in relationship to their pathogenicity, one aspect that has been largely ignored is its ability to lower the pH of the medium, via the secretion of oxalic acid or other low molecular weight organic acids, which is necessary for their ability to colonize or cause infection. Oxalic acid is a known pathogenicity factor of plant fungal pathogens. This acid is secreted in the host tissues and accumulates in the form of oxalate, leading to a pH decrease that stimulates the production and activity of fungal enzymes. Moreover, as oxalate is a strong chelator of divalent metallic cations, it can sequester calcium ions, with different possible structural and physiological consequences for the host.
Given the importance of oxalic acid production in plant pathogens, this compound might also play a role during human infection by opportunistic fungal pathogens. In fact, several studies have reported the presence of calcium oxalate crystals for pulmonary aspergillosis, and the detection of calcium oxalate crystals has been proposed as an easy tool for differential diagnosis. In most of the reported cases, oxalate deposition is associated to A. niger infection, but some reports also include infection caused by A. flavus or A. fumigatus. Oxalic acid and oxalate crystals are thought to cause damage to host tissues (including pulmonary blood vessels), as well as tissue injury via iron-dependent generation of free radicals. Aside from mechanical damage to the host tissues, the formation of calcium oxalate could also have a dramatic effect on cell physiology. Indeed, calcium is a secondary messenger in many cell types, including those of the immune system. During immune stimulation, Ca2+ entry from extracellular medium or cellular compartments increases intracellular Ca2+ concentration, and thus Ca2+ chelation has been shown to inhibit the immune response in vitro. All this suggests a role of oxalic acid also in the inhibition of the immune response. Despite these converging indications, a link between oxalic acid production and pathogenicity for Aspergillus spp. in animals has only been recently demonstrated.
The importance of the lung microbiome
For a long time considered sterile, the lung is now known to harbor a microbiota composed of bacteria, fungi, and viruses. This microbiota has a different composition in a healthy state, in the presence of respiratory diseases, and after transplantation. Moreover, dynamic intra-individual variations accompany changes in health status. Although most studies in this area are descriptive, recent data provide clear evidence for the role of the airway microbiota, for instance, in the modulation of the host immune response or mucus production. It can be expected that the lung microbiota also plays a role in aspergillosis. However, many knowledge gaps still exist regarding the link between the pulmonary microbiota and aspergillosis. For instance, alteration of the air-blood barrier, particularly access to the extracellular matrix, is a risk factor for fungal infection, notably in the case of Aspergillus. Therefore, revealing the impact of the pulmonary microbiota on the strengthening of this barrier is of clinical importance. Also, the coexistence of fungi and bacteria, and interactions with the host, have only partially understood implications for nutrient acquisition[AB1] . Therefore, integration of principles from ecological theory, including the understanding of cooperation or antagonism between fungi, bacteria and their human host, has high potential: this knowledge makes it possible to identify manipulable elements from within the airway microbiota for the development of therapeutic tools to control Aspergillus.
From soil ecology to Aspergillosis, Laboratory of Microbiology, UniNE
A. fumigatus, is typically a soil-dwelling saprotrophic fungus. Therefore, our current understanding of its ecology in soils can be a source of inspiration for finding new ways to control its pathogenic potential in the human environment. Although comparing soil ecology and human health may appear far-fetched, important analogies can be drawn between the two systems. As indicated above, one of the hypotheses leading to this project is that Aspergillus spp. use secretion of oxalic acid as a pathogenicity factor. We have previously investigated the metabolism of oxalate in soils, where consumption by heterotrophic bacteria (oxalotrophic bacteria) is the main sink of oxalate. Based on our knowledge in soil ecology, we have identified considerable parallels between oxalate production/consumption in soils and the human body. In both cases, the sources of oxalate are multiple but the sink remains oxalotrophic activity. Moreover, a comparison of the known oxalotrophs in soils and the composition of the human microbiota points to the abundance of a diverse community of oxalotrophic bacteria at least in the human gut.The integration of oxalate metabolism and fungal pathogenicity is made through the principle of environmental interference. According to this novel principle, by the secretion of oxalic acid, the pathogen manipulates abiotic parameters in the hosts, mainly pH and Ca+2 concentration. Thus, the consumption of fungal oxalate by oxalotrophic bacteria regulates pH and inhibits colonization.
We conducted a series of tests, based on in vitro confrontations to verify this principle. Most relevant of all for this project, experiments performed with the soil oxalotrophic bacterium Cupriavidus oxalaticus and the pathogen A. niger on differentiated bronchial tissue in transwells and bronchioles-on-a-chip (BoC) systems demonstrate the validity of the environmental interference principle. In these experiments, we showed that A. niger triggers a cytopathic effect. Furthermore, conidial germination and fungal growth resulted in the acidification of the culture media as predicted by our model. This correlated with a decrease in the calcium concentration in the culture supernatants, and a reduction in the concentration of free oxalic acid. The first three observations were explained by the formation of oxalic acid crystals in the medium. Each of these parameters returned to the baseline physiological levels of the lung cells alone when the biocontrol bacterium was co-cultured with the fungus. Likewise, crystal formation was totally suppressed. Overall, the results validate the underlying hypothesis according to which oxalotrophic bacteria can be used to manipulate the microenvironment created by A. niger to arrest its growth. The next step required to translate these results into a clinical application consists in a validation in an in vivo model as proposed in this project. Moreover, given that the oxalotrophic bacterium caused an increase in lactate dehydrogenase (LDH) leakage as a proxy for cellular damage (expected as this soil bacterium is foreign to the lung environment), finding new oxalotrophic bacteria from within the airway microbiota is essential to develop a biocontrol approach compatible with the human ecosystem.
Pulmonary microbiota of lung transplant patients as a longitudinal tracking model – Division of Pulmonology/CHUV
Bronchoalveolar lavage fluid (BALF) collected through bronchoscopy is a key clinical and research tool allowing sampling of the small airways and the alveoli. Due to the semi-invasive nature of this approach, healthy subjects are rarely analyzed, and ill patients are rarely followed up consecutively. Lung transplantation stands out from these limitations, thanks to the routine post-transplant bronchoscopic monitoring of recipients. Since 2012, our center has followed up nearly 100 patients with an average of 5 BALF samples per patient. These samples allowed us to investigate the dynamics of the bacterial lung microbiota, typically between 0.5 and 48 months after transplantation, and to demonstrate important correlations with different gene expression profiles in host innate immune cells, including a strong link with the level of inflammation. In addition, combining observations in patients with in vitro experiments highlighted the potential impact of the microbiota on the level of extracellular matrix deposited in the lung. Taken together, these observations show that the pulmonary microbiota may play an important role in the integrity of the air-blood barrier. A key next step in this research, as developed in this application, will be to determine the implication of pulmonary microbiota in the risk of fungal infection.
In the transplanted lung, we have recently identified a balanced and stable community of prevalent bacteria, dominated by the genera Prevotella, Streptococcus and Veillonella, globally associated with a favorable clinical status. This contrasted with two other communities dominated respectively by the much less prevalent Staphylococcus and Pseudomonas genera, both of which were associated with a suboptimal control of local and peripheral host immunity, a higher risk of infection and impaired lung function. Interestingly, preliminary transcriptome analysis of BALF innate immune cells showed that the balanced bacterial community and the two disrupted communities were associated with different gene expression related to control of the air-blood barrier, inflammation and the metabolism of iron and calcium. These observations establish a link between airway bacterial communities and host metabolic pathways involved in the control of oxalic acid-dependent Aspergillus infection, paving the way for testing the therapeutic manipulation of the lung microbiota.
Bioinformatics and data mining in the lung microbiome - SIB
The SIB is a world class bioinformatics institution that has contributed to the early developments of in silico methods for the automated annotation of protein sequences promoting the use of Generalized Profile technology (https://github.com/sib-swiss/pftools3). More recently, the Vital-IT group is leading the development of MetaNetX, a public resource devoted to Genome-Scale Metabolic Networks (GSMN) that computes a large reconciliation of metabolites and biochemical reactions from public external resources, now widely used in the field of Systems Biology. This has led to the development of new numerical methods to integrate and interpret "omics" data, including a new algorithm to make sense of RNA-seq data with the help of a GSMN. Of particular significance here is a previous collaboration with Dr. Philippe Hauser (CHUV) on Pneumocystis jirovecii, an unculturable opportunistic fungal pathogen of the human lung. The genome of P. jirovecii was assembled from a single bronchoalveolar lavage, making it possibly the first eucaryote genome assembled from a clinical sample. Subsequent (meta-)genomics studies on this organism contributed to the understanding of its biology and led to the recent description of the molecular mechanisms of its antigenic variations, that suggests that P. jirovecii utilizes a hitherto undescribed strategy to escape the immune system.
Up to date, the characterization of the oxalotrophic potential based on the metagenomic data comparison between the upper respiratory tract (mouth and nose) and the pulmonary and gastric microbiotas of healthy individuals has not been possible. Therefore, there is a clear need for better data quality and bioinformatic methods to assess the oxalotrophic potential of the native human lung microbiome.
Organ-on-a-chip and next generation tissue systems – AlveoliX and SiMPLInext
The current crisis in the efficiency of pharmaceutical research and development has driven the generation of novel in vitro models that better mimic in vivo conditions. This development goes hand in hand with the debate concerning the ethics of animal experimentation and the development of alternatives focused on animal welfare. In this area, AlveoliX is one of the pioneering companies that has developed an easy-to-handle lung-on-a-chip (LoC) array that integrates the key feature of mechanical breathing and enables simple sampling of the culture supernatant and visualization of the cells. AlveoliX will provide the system and chips as well as support and know-how required for achieving the scientific plan.
SiMPLInext, the second key industrial Swiss partner, provides next-generation experimental platforms with relevant physiology and reliable quantitative scoring based on proprietary organ-on-chip technologies. SiMPLInext, is developing, industrializing and marketing a new generation of a connected complementary toolset for qualifying human tissues produced in vitro and quantitatively monitoring their alteration under the action of external stimuli. Target organs are those, such as the lung, that contain structures called biological barriers associated with absorption mechanisms related to drug toxicity and efficacy. The high throughput capacity of this system combined with real-time monitoring of the integrity of a reconstituted air-blood barrier makes this system the optimal option for screening experimental conditions, and for prospective developments including addition of immune cells and bacterial consortia. Cross-validation of the complementary data generated by the different experimental systems used in our project will be invaluable for their future commercialization.
In this project, we will use the extensive knowledge gained on oxalotrophy in soils and in the microbiota of lung transplant recipients as steppingstones for the understanding of the potential of the human airway microbiome in controlling the development of fungi from the genus Aspergillus via the regulation of the concentration of oxalic acid secreted by the pathogen. This will lead to the discovery of new bacterial candidates for the development of therapeutic targets. We have provided a proof-of-concept of the use of oxalotrophic bacteria to control the growth of A. niger and this represents a novel paradigm in which the host environment is part of the focus of the medical intervention in the fight against opportunistic fungal pathogens. In sum, this project will translate this proof of concept into a demonstration of clinical relevance and strengthen our initial approach by complementing oxalotrophy-based biocontrol with biocontrol targeting a reinforcement of the air-blood barrier. Accordingly, the aims of this project are:
To demonstrate the in vivo potential of environmental interference in a murine model to validate the clinical relevance of the developed principle as a complementary approach to traditional treatment of fungal infection.
To isolate new biocontrol airway bacteria with the ability to degrade oxalic acid and/or reinforce the air-blood barrier. The expected biocontrol should be as effective as that obtained with the soil bacterium used in the proof-of-concept experiment, but without adverse side effects. The result will be a collection of organisms allowing the development of therapeutic options through transplantation of oxalotrophy or other biocontrol function into an airway microbiota that lacks these functions, in a similar way to the transplantation of fecal flora is becoming a therapeutic approach in chronic cases of Clostridium difficile infection.
To implement bioinformatic tools for clinicians aimed at the identification of at-risk patients based on the screening of the airway lung microbiota. This will be achieved by combining the development of bioinformatic resources for the functional characterization of oxalotrophy with the extensive knowledge gained from transplanted patients and the bank of samples and data from transplanted patients. To reach this aim, we will once more translate soil ecology into medicine by extrapolating the concept of disease-suppressive versus conducive soils57, which links the composition of the soil microbiota with the natural protection against fungal plant infection. The goal is to apply the same concept to infection in humans by testing the hypothesis that the composition and functional characteristics of the airway microbiota influence the establishment of infection.
To accomplish this ambitious scientific agenda, we parallelize the work tasks, efficiently combining five methodological approaches to bridge computational prediction with in vitro testing and relevant in vivo models. This is achieved through an academic-industrial partnership to connect the traditionally long path from the in vitro testing to the in vivo animal models, via the use of high-tech qualification and screening in advanced 3D cultures with classical model architecture (SiMPLInext technology), together with a physiologically relevant breathing lung-on-a-chip (LoC) system (AlveoliX technology). In this project, an innovative use of both technologies will be implemented by demonstrating their application for the fast screening of candidate biocontrol bacteria for both individual strains and complex microbial communities. With this, we will provide a case example of the power of combining organ-on-a-chip technologies with state-of-the-art bioinformatics tools and organismal models at the forefront of medical research in the field of fungal pathogenesis. Moreover, by cross validating infection risk indicators and most effective biocontrol bacteria across a large spectrum of experimental approaches, we will develop not only a unique set of tools for the personalized care of patients most vulnerable to aspergillosis, but also a solid experimental platform to assess the potential of novel therapeutic approaches applicable in the field of fungal pathogens.
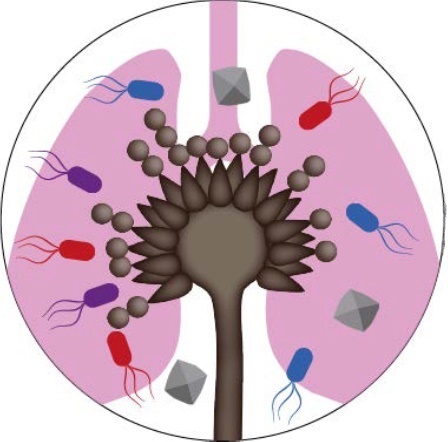